Research
Solar Cells
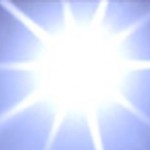
We seek to supersede traditional optoelectronics with devices that use excitons to mediate the flow of energy. Mastering the properties of excitons offers the ability to guide energy at the nanoscale, and transform it with a flexibility that is impossible in conventional systems. For example, excitons have no net charge and can be split or combined. As an example, we have exploited this flexibility by using singlet exciton fission to generate multiple carriers in a solar cell at an efficiency of > 1.26 electrons per photon, exceeding the unity limit in conventional electronic materials.
The spintronics of excitons is key to our work. Indeed, the high efficiencies of singlet exciton fission arise because competing thermalization losses are spin disallowed.
Figure: Unlike electrons, excitons can be split. In the Center for Excitonics, we have used singlet exciton fission in pentacene to generate at least 1.26 electrons per photon. From Baldo, Van Voorhis, et al. Science (2013) and APL (2013)
Photovoltaic (PV) cells remain an expensive source of electrical power. We believe that the best way to reduce the cost of PV energy is to improve efficiencies. This reduces both the cost of the solar cell per Watt generated, as well as reducing the installation cost, since fewer cells are required.
We are following two approaches to reducing the cost of PV energy.
(i) Solar antennas
Solar antennas are optical devices installed as coatings on solar cells or as separate light absorbing structures. They can be used to concentrate light or enhance efficiency. For example, we have built solar concentrators that collect light using organic dyes and concentrate it on solar cells. The architecture is known as a luminescent solar concentrator (LSC). It enables high optical concentration without excess heating in a stationary system. Perhaps the ultimate LSC is a solar powered laser. Here, sunlight is transformed into a coherent beam. This allows the maximum possible optical concentration, and can also be used for wavelength conversion. Finally, we have been investigating the use of singlet exciton fission in solar antennas. Exciton fission can occur in organic materials when the singlet exciton has almost exactly twice the energy of the triplet. We are interested in using this mechanism to improve the efficiency of inorganic solar cells in the visible spectrum.
See our work on:
- Organic dye-based Luminescent Solar Concentrators
- Glass-based Luminescent Solar Concentrators
- Solar powered lasers
- Singlet fission-based antennas
(ii) Organic Solar Cells
Organic solar cells promise to be cheap sources of photovoltaic energy. They presently limited, however, by relatively low efficiencies. One notable aspect of organic cells is that their operation is much closer to photosynthesis than conventional photovoltaics. Our initial work employed biological materials extracted from plants and photosynthetic bacteria. This was perhaps the first demonstration of the integration of photosynthetic protein-molecular complexes in solid state devices. More recently, we have continued our interest in photosynthesis by imitating the multistep charge dissociation pathway. This led to a focus on the charge transfer states that mediate both exciton dissociation and charge recombination. Our other focus in organic photovoltaics is spin engineering. We are following the example of organic LEDs, where control over exciton spin led to large increases in efficiency. In principle, control over spin offers three benefits: much longer exciton diffusion lengths for triplet (spin 1) excitons, the ability to turn off recombination by engineering triplet CT states, and exploiting singlet exciton fission to increase photocurrents. See our work on: